Biomedical Engineering
The revolution from the test tube
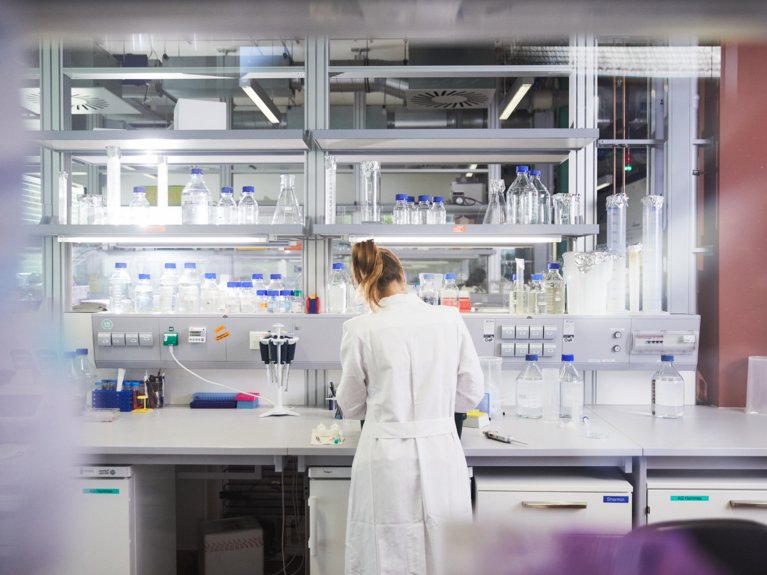
Laboratory at the Max Delbrück Centre in Berlin. Picture: Katharina Bohm / Max Delbrück Center
Just as engineers changed our outer world with the findings of physics in the last century, bioengineers are on the cusp of transforming our inner world with the tools of biomedicine in this century. This will fundamentally change the way we recognize and treat diseases.
The last century is considered the age of physics. Engineers used the fundamental findings to develop technical applications that changed the world. From the development of computer hard drives to GPS and the prospect of self-driving cars. "This century will be about mapping, modifying and controlling our inner world: Genes, tissues, organ systems and ultimately the human brain." So says Michael I. Miller, head of the Department of Biomedical Engineering at Johns Hopkins University, one of the leading experts in the field of biomedical engineering. And numerous researchers agree: The term represents nothing less than a revolution in science and medicine. A revolution that has already begun and whose potential is far from exhausted.
A few examples: The CRISPR-Cas9 gene scissors, which make it possible to specifically alter gene sequences in living cells in order to treat diseases or make crops more resilient to climate stressors and more productive at the same time. Or even innovative forms of therapy: A drug that is no longer absorbed by the entire body tissue and may have unwanted side effects, but only works where it is supposed to. As if it had been given a specific address before being taken.
With the CRISPR-Cas method, sections of DNA can be specifically removed, inserted or switched off. Image: Sergey Nivens7Shutterstock
But what does bioengineering actually mean? It is an interdisciplinary field in which scientists, engineers and doctors make use of the findings from basic research and develop medical or biotechnological processes. A clear-cut definition is difficult. This is also due to the research field itself. Bioengineering is a best-of from various research areas that at first glance do not appear to have much in common, such as materials science, genetics, physics, bioinformatics and cell biology. Beyond the interdisciplinary approach, the practical application is crucial. "The aim is to combine knowledge and tools from these fields in such a way that the newly developed technologies can be applied to biological systems. Whether in medicine, agriculture or environmental protection, bioengineering is extremely solution-orientated," says Maike Sander. The 56-year-old heads the Max Delbrück Center in Berlin. The researcher has been working in bioengineering for over three decades, spending a lot of time at renowned research institutions in the USA and experiencing first-hand how research, teaching and industry can benefit from it.
Maike Sander. Picture: Peter Himsel / Max Delbrück Center
When Sander returned to Germany in 2022 to take over the management of the Max Delbrück Centre, she was surprised at how little the term bioengineering is used in this country compared to the USA or the UK. There were no separate departments here, no Bachelor's or Master's degree programs. Instead, it was a pretty blank spot on the research map, despite the fact that everything is in place to play the field.
Sander is optimistic that this gap can be closed very quickly. On the one hand, appropriate investment must be made in training and infrastructure. On the other hand, institutions such as the Helmholtz Association can already make a significant contribution. "We have everything it takes because we are extremely interdisciplinary. We are not just about medicine or biology. We have institutes such as DESY in Hamburg and the Helmholtz Centre Berlin, where physicists and engineers work with particle accelerators. In Dresden-Rossendorf, we are researching new materials. The main task now is to link these individual areas together."
The recently established Biomedical Engineering Taskforce will help with this. It uses the expertise and infrastructure of a large number of Helmholtz research centers beyond the field of health to further advance the "biomedical engineering revolution" and better connect research and industry. Over the next few years, six core projects will be developed from concept to market-ready application.
One of the projects is dedicated to the development of new molecular diagnostics for the early detection and prevention of widespread diseases such as cardiovascular diseases, cancer, dementia and diabetes. Ideally, biomedical and AI-supported multi-omics methods can be used to analyze many different types of biological samples at several levels, for example, from genomics (DNA), proteomics (proteins) or metabolomics (metabolic products). This means that new diseases or relapses can be recognized much earlier than before, possibly even before clinical symptoms appear.
CAR-T cells (green) attack lymphoma cells (red). In CAR-T cell therapy, immune cells taken from cancer patients are upgraded in the laboratory so that they recognise certain surface proteins of the malignant cells. Picture: AG Höpken / Rehm, Max Delbrück Center
In another project, the methods of synthetic biology are being used to further develop immune cell therapies. "Let's imagine cancer treatment," explains Maike Sander. "We are now able to modify the patient's own immune cells so that they behave in a certain way and actually only fight the cancer instead of causing undesirable side effects."
The possibilities of biomedical engineering seem almost limitless. "Helmholtz Biomedical Engineering's mission is simple but bold: To pioneer solutions that improve health," says Maike Sander. "The Helmholtz Association is ideally positioned to bring together the necessary expertise from different research fields as well as academic and industrial partners to push the boundaries of interdisciplinary innovation. The initiative will work along the entire translational value chain, from building new knowledge about biological systems, to prototype development and validation, to clinical trials. This will create the urgently needed new solutions for earlier disease detection, precise prevention and improved therapies."
However, one person's wishes are often another person's fears. Sander knows from experience that skepticism towards innovative technologies, particularly in the field of synthetic biology, is greater in Germany than in the USA. This is another reason why she is in favor of a transparent and ethically and morally sound approach to this field of research. Only then will it be possible to realize the full potential of the bioengineering revolution.
Readers comments