CRISPR/Cas
Shotgun vs. scalpel
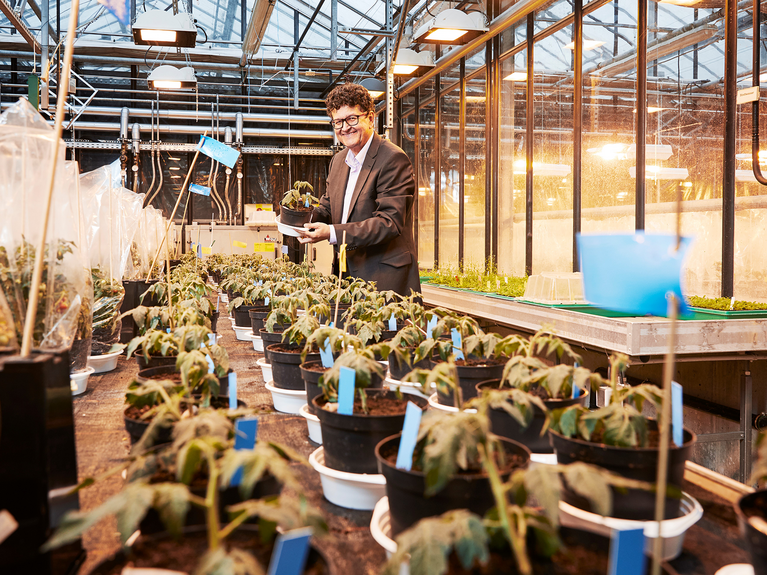
Holger Puchta with genetically modified tomato plants in the greenhouse of the Joseph Gottlieb Kölreuter Institute of Plant Sciences (JKIP) at the Karlsruhe Institute of Technology (KIT). Image: Sandra Göttisheim/KIT
Molecular biologist Holger Puchta has been researching modern plant breeding methods for years. Genome editing is faster and more precise than conventional mutagenesis. He therefore welcomes the EU Commission's proposal to relax restrictions on genetic engineering methods in plant breeding.
When Holger Puchta wants to illustrate the enormous genetic potential of a single plant species, he refers to Brassica oleracea, better known in horticulture as wild cabbage. This is because the herbaceous wild plant with the yellow flowers is the ancestor of a whole range of vegetables that have provided varying degrees of pleasure on the plates of generations of children. White cabbage and kale, cauliflower and Brussels sprouts, broccoli and kohlrabi all descend from the inconspicuous cruciferous plant.
Many well-known cabbage types are cultivars that can be traced back to a common cabbage species. Image: Stockcreations/Shutterstock
"It's amazing what classical breeding has teased out of this one species," says Holger Puchta. But all that took time. A lot of time. The breeding history of wild cabbage goes way back to ancient times, and probably beyond. "We obviously don't have centuries or even decades if we want to counter the consequences of climate change and continue to feed a growing world population," explains the molecular biologist. "We need agricultural crops that are as salt, heat and diseaseresistant as possible, high-yielding, and flavorful. And we need them fast. The standard tools of classical breeding and mutagenesis work too slowly. With molecular scissors, we can now do it within one or two plant generations, within months."
Holger Puchta counters reservations about this technology with a practical look back at the past: "You have to realize how breeding was done before gene shears and how it still is. Mutagenesis has been common and legal practice for about 70 years. In this process, radioactive irradiation creates 10,000 uncontrolled mutations in a plant. By chance, a desired result then sometimes emerges." In contrast, molecular scissors create a single mutation in a very specific gene in a very targeted way. "So we have mutagenesis and thousands of uncontrolled mutations on one side, and molecular scissors and a single targeted change on the other. It’s like having a shotgun and a scalpel. The latter is faster, safer and highly specific," says Puchta.
In these tomato plants, the molecular scissors CRISPR/Cas were used to modify the genome. Image: Sandra Göttisheim/KIT
Since 2012, a very specific gene-scissors methodology has been used worldwide for this purpose: CRISPR/Cas, the development of which earned scientists Emmanuelle Charpentier and Jennifer Doudna the 2020 Nobel Prize in Chemistry. "Through CRISPR/Cas, we are currently experiencing the biggest revolution in 30 years in biology, medicine and agriculture, because the method is extremely flexible and accurate," says Holger Puchta. At the Karlsruhe Institute of Technology (KIT), he has significantly expanded the possible applications of CRISPR/Cas and, for the first time, lifted it from the level of individual genes to the level of the chromosome.
Genes are arranged linearly on chromosomes. For a long time, CRISPR/Cas was only used to change individual genes on a chromosome. A few years ago, however, Puchta and his team succeeded in exchanging entire parts of chromosomes and thus recombining the genes. Thanks to this breakthrough from Karlsruhe, it is now possible to, among other things, separate good properties of a plant from bad ones. If, for example, in species X a gene for a bitter substance that makes the plant's fruit taste bad is located directly next to a desirable gene that makes it resistant to a virus, both genes will always be inherited together because of their immediate proximity on the chromosome. Therefore in the normal generation sequence, all plants with resistance will always also be bitter. With Puchta's technique, the chromosome part with the virus resistance can now be separated, transferred to another chromosome without bitter and then inherited separately.
Also using CRISPR/Cas, Holger Puchta's research group succeeded in solving a similar problem. If genes for positive traits are located far apart on a chromosome, they are usually separated from each other during inheritance and are thus gradually lost during breeding. Using molecular scissors, KIT researchers managed to cut out most of a chromosome, turn it 180 degrees, and reinsert it. "In this way, we shut down almost a complete chromosome and make it virtually invisible for gene exchange with other chromosomes," explains Holger Puchta. "The positive properties on the inverted section are thus fixed, and will in the future always be inherited together as a package, and will therefore be preserved."
Holger Puchta in the air-conditioned cultivation room in the basement of the JKIP at KIT. Image: Sandra Göttisheim/KIT
The research work from Karlsruhe has helped to significantly increase the toolbox in plant breeding. "We now know very precisely which genetic changes are necessary to produce larger and a greater quantity of fruits, for example, or to reduce bitter substances in order to improve the taste," says the KIT scientist. "With the new methods, we can thus transform, for example, a heat and salt resistant wild species from warm and dry regions like Africa into a high-yielding and tasty crop for Europe's agriculture practically in fast motion. We urgently need such plants, which can withstand the effects of climate change and reduce the use of pesticides thanks to additional disease resistance."
This year, Holger Puchta's research at KIT received a 1.22 million euro grant in a Reinhart Koselleck project of the German Research Foundation (DFG), the DFG's highest endowed excellence funding for individuals. And even politicians, who have long been skeptical, are increasingly recognizing the value and necessity of the technology. For example, the EU Commission wants to re-regulate the use of genome editing methods such as CRISPR/Cas in plant breeding and place new plant varieties created in this way on an equal footing with conventionally bred plants under certain conditions. "This makes perfect sense from a scientific point of view," says Holger Puchta, who has also advised policymakers for years. "With genome editing, we don't introduce foreign genetic material into plants. The technique is fast, precise and safe. And it would be a bit strange to allow mutagenesis, the uncontrolled shotgun, and ban the scalpel. Incidentally, neither method has ever turned a plant into a monster."
Readers comments