Quantum Year 2025
Part #05: Quantum materials with a twist
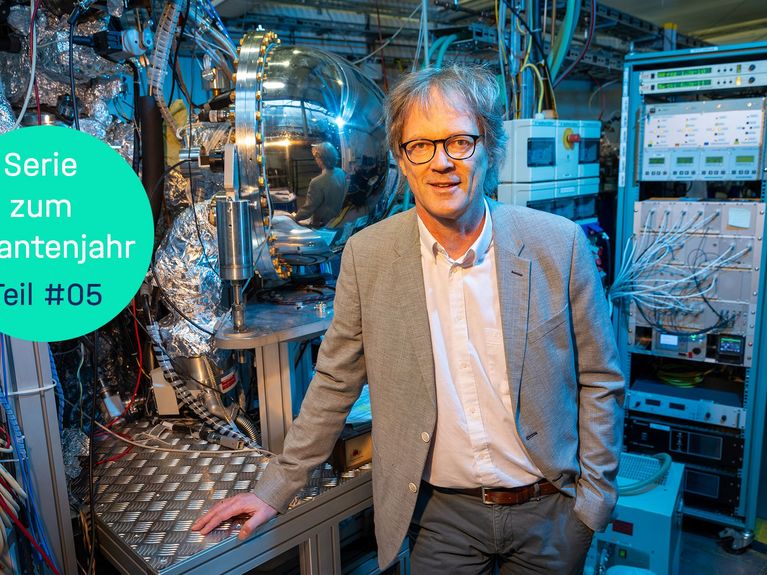
Picture: HZB
In the fifth instalment in our series for the Quantum Year, we spoke with solid-state physicist Oliver Rader from the Helmholtz-Zentrum Berlin (HZB) about topological insulators – a quantum mechanical wonder material.
The birth of quantum mechanics 100 years ago was preceded by groundbreaking experiments. One of them was the famous Stern-Gerlach experiment from 1922, in which a narrow beam of silver atoms was passed through an inhomogeneous magnetic field. When the silver atoms hit a screen, they didn’t form a coherent spot, as would be classically expected. Instead, they tended to split in two directions, which manifested in two frequency distributions. This seemingly strange result can ultimately be explained by spin in quantum particles, a kind of quantized intrinsic angular momentum similar to a spinning top rotating around itself. In the Stern-Gerlach experiment, it is the spin of the outermost electron of the silver atom and its directional quantization in the inhomogeneous magnetic field that is responsible for splitting the beam in two.
Almost three quarters of a century later, fundamental findings on orbital and intrinsic angular momentum – the so-called spin-orbit coupling of electrons – formed the point of departure for developing the topological insulator, a completely new type of material. “The topological insulator represents a special class of materials because it is characterized by unique electronic behavior,” as Oliver Rader explains. On the inside, the insulator is non-conductive, i.e., insulating, for electric current. On its surface, however, current can flow virtually loss-free – a bit like a coffee can, although the topological insulator is made of a homogeneous material. “In this context, we’re talking about material properties that retain their stability even if the underlying material undergoes mechanical or certain chemical changes.” In the case of topological insulators, these special properties are linked to certain electron paths on the surface, which are protected by the “topology,” i.e., the structure. The first topological insulator was made using a thin layer of mercury telluride. This suddenly opened up the prospect of completely new technical applications, from virtually loss-free electricity transport in the field of information technology to use in quantum computing. In the years since, dozens of chemical compounds with these electronic properties have been produced in the laboratory. However, their production and handling are still extremely challenging.
Current transport on the surface of a topological insulator is particularly robust and involves lower losses than with conventional materials – behavior that can only be understood in terms of quantum mechanics. “Topological insulators are therefore also referred to as quantum materials,” says Rader. These materials are characterized by the strong coupling of different physical degrees of freedom. In the case of topological insulators, this is primarily the quantum mechanical effect of spin-orbit coupling. “That is, the interaction of the orbital angular momentum of an electron with its spin,” Rader explains. An electron’s direction of movement on the surface of the topological insulator determines its spin. It is practically quantum mechanically entangled with other degrees of freedom in the quantum system. Accordingly, electrons that move in opposite directions also have opposite spins. “In other words, an electron cannot simply change its direction of movement without also changing the orientation of its spin.” Under special conditions, this leads to the complete suppression of spontaneous changes in direction or backscattering of electrons in the topological insulator. “What we’re talking about here is topological protection, which the electrons are subject to in this way,” says Rader. “However, in order to exploit these effects for electronic components, the topological insulators must first be magnetized, or more precisely, turned into permanent magnets.” For many years, this was achieved simply by mixing atoms with a magnetic moment into the insulators. “The components produced a sensational quantum effect, but only worked at very low temperatures due to the resulting disorder in the material.” Rader’s group then discovered a new class of materials with manganese bismuth telluride – which made it possible to create what are known as intrinsic magnetic topological insulators. Here, the magnetic atoms create a completely new and regular crystal structure.
How did they make their discovery? “Research into quantum materials requires an intensive interplay of theory and experimentation. And a lot of patience,” says Rader with a laugh. Healthy competition can help, too. “We critically examined the supposed success stories of other research groups and soon realized that their results weren’t correct.” Rader’s group then replaced a previously used element called selenium with another with a higher spin-orbit interaction: tellurium. “That solved several problems at once. And by using specific magnetic atoms, we were able to create an ordered chemical structure within the material.” In experimentation, the researchers have to employ various methods to investigate the problem comprehensively. For example, Rader’s group uses photoelectron spectroscopy, in which sample materials are illuminated and examined with the soft X-ray light produced at the Berlin synchrotron radiation facility BESSY II.
Magnetic topological insulators now represent a promising basic concept for new types of electronic components that function more effectively and energy-efficiently than conventional technologies. According to Rader: “The overarching term for this type of electronic application is spintronics, a portmanteau of spin and electronics, in which the spin of electrons is used for information processing and storage in addition to the electrical charge.” Fields of application can also be found in quantum metrology and quantum sensor technology, where quantum effects are used to improve measurement accuracy in specially designed measuring devices. However, magnetic topological insulators are also highly interesting as potential component platforms for qubits in quantum computing. “This is also due to the fact that, as mentioned, they are particularly resistant to external interference and influences, which makes them extremely attractive for use in quantum computers. However, this would require so-called topological superconductors.” Though such superconductors don’t yet exist, Oliver Rader is convinced that we’re not that far away from being able to produce them.
We’ll revisit this topic in the next instalment. Stay tuned!
Readers comments