Quantum electrodynamics
Eavesdropping on the void
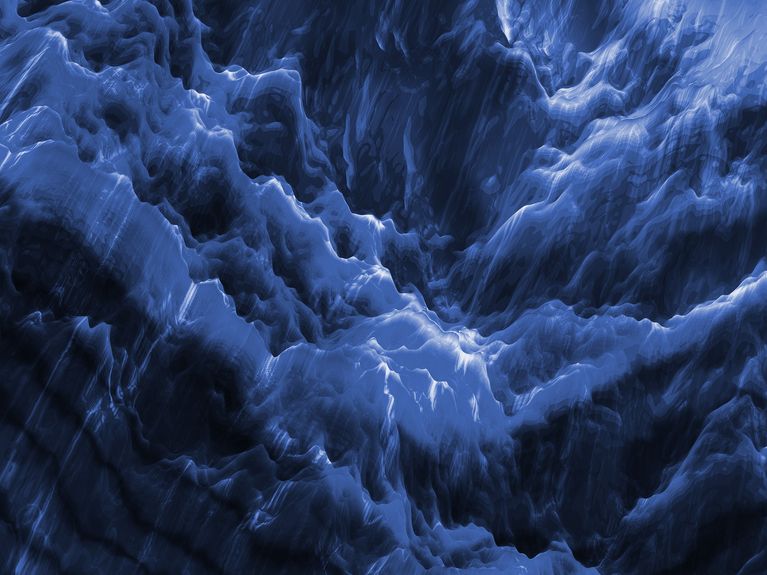
Illustration (colored): Zita/Shutterstock
New experiments will help to investigate the mysterious structure of the quantum vacuum. The Helmholtz-Zentrum Dresden-Rossendorf is involved in the design and implementation.
Nothing is simpler than nothing, you might think. Because where there is nothing, all complexity should be reduced to zero. But modern physics contradicts this. “The so-called quantum vacuum is by no means just the absence of matter; the vacuum itself is the carrier of the laws of nature and thus also a kind of ‘primordial substance’ from which the existence of all elementary particles can arise,” says Ralf Schützhold, Professor at TU Dresden and Director of the Institute for Theoretical Physics at the Helmholtz-Zentrum Dresden-Rossendorf (HZDR).
In other words: The vacuum, as it is imagined in modern physics, is not simply a void, but has structure. This sounds rather philosophical, but it is one of the central statements of modern physics. “In our world, the famous Heisenberg uncertainty principle applies,” explains Schützhold. “According to this principle, there is a certain uncertainty everywhere in nature, including in a vacuum, i.e., a limit to what can be precisely determined.” An absolutely empty space that contains neither particles nor electromagnetic fields does not exist, it is always filled with tiny quantum fluctuations. This applies in particular to the electromagnetic fields described by quantum electrodynamics, the most precisely tested theory in physics.
“There are different ways of visualizing this abstract concept of quantum fluctuation,” says Schützhold. “You can call it a ‘quantum flicker’ because the quantum fields undergo fluctuations in a very small space and on extremely short time scales that cannot be explained classically.” There is also often talk of ‘virtual particles’, which are created in a vacuum and disappear again practically instantaneously.
Ralf Schützhold is Director of the Institute for Theoretical Physics at the Helmholtz-Zentrum Dresden-Rossendorf. Photo: HZDR/André Wirsig
The exciting thing is that it is possible to manipulate the state of the vacuum. For example, if you bring two electrically conductive plates very close together, only certain quantum fluctuations can form between them, whereas other quantum fluctuations which occur in free space, can no longer. The difference between the quantum fluctuations inside and outside the plates creates a tiny force that presses the two plates together. “This force is known as the Casimir effect after its discoverer and plays an important role in nanotechnology, among other things,” says Schützhold. “We now want to demonstrate a related effect, although we are working with laser beams and not with conductive materials.”
Together with colleagues at the European XFEL near Hamburg, the world’s largest and most powerful X-ray laser, the HZDR researchers are setting up a special experiment that is one of the Helmholtz Association’s flagship experiments due to its pioneering technology and significance for physics. The European XFEL makes it possible to test quantum fluctuations with extremely high field strengths. The idea behind the experiment is as follows: An extremely strong, optical laser pulse is shot into a vacuum chamber and focused very sharply on a single point. Enormous electromagnetic field strengths can be achieved at this focal point, which polarize the quantum vacuum. “We want to get the quantum flicker to vibrate preferentially in one direction, so to speak,” says Schützhold.
The X-ray beam from the world’s largest X-ray laser, the European XFEL, only becomes as clearly visible as in the photo in complete darkness and with an exposure time of 90 seconds. In 2024, the first experiments to detect quantum fluctuations in vacuum will take place here. Photo: European XFEL/Jan Hosan
An X-ray pulse should then pass through the focal point of the optical laser beam at the same time. Such an X-ray pulse contains around one trillion X-ray photons. “In this experimental setup, approximately one of these one trillion X-ray photons should have undergone a measurable change in polarization per pulse due to the influence of the polarized vacuum, which we want to prove with the help of polarization filters,” says Schützhold. “This means that we are pretty much at the limit of current measurement technology for detecting this extremely weak effect.”
In order to simplify the verification process, the scientific community is also considering alternative experimental set-ups. “We are considering using two optical lasers instead of one and shooting the two laser pulses against each other at an angle,” says Schützhold. Because when two waves run against each other, a so-called standing wave is created. This would create a grid-like electromagnetic field. This also polarizes the vacuum. The researchers then also want to “interrogate” its changed properties with an X-ray pulse. Similar to a crystal lattice, in which X-rays are diffracted – i.e., deflected – a quantum vacuum oscillating in the form of a lattice could also deflect the X-ray photons, at least very slightly.
X-ray photons are not fundamentally different from optical light. Both are electromagnetic radiation, but X-rays have higher energy. If this experiment works, we would have proven that light is scattered by light. The additional deflection of the X-ray photons makes it much easier to filter them out of the original X-ray pulse, which should significantly simplify detection. The first preparatory tests are due to start as early as 2024, with actual measurement operations scheduled to begin in the coming years.
“These experiments are not just about testing the limits of the technology,” emphasizes Schützhold. “We want to use such experiments to study the properties of the quantum vacuum and see whether our current theories are correct.” The current theory of elementary particles – the so-called standard model of particle physics – is considered to be complete. All the particles predicted by the standard model have already been proven experimentally. The last outstanding particle was the Higgs boson, which was found at CERN in 2012.
Nevertheless, there are still unsolved problems and unanswered questions. Particle physicists have long been pondering what dark matter in the cosmos might be all about. Although it has significantly more mass in the universe than our ordinary matter, experiments have not yet produced the slightest indication of what kind of matter dark matter might be. “If we shine a light through the quantum vacuum, with a bit of luck we could find unexpected effects that are not predicted by the standard model of particle physics,” says Schützhold, describing the hopes of the research community. Previously undiscovered particles could be behind the quantum fluctuations, for example, ultra-light ghost particles called axions. “And that,” says Schützhold, “would be a clear sign that there are other, previously unknown laws of nature.”
The discrepancy between theory and experiment should then allow certain conclusions to be drawn about the new particles or laws of nature, such as the nature of dark matter. But even if such a sensation fails to materialize, the results are still valuable, as certain theoretical models of dark matter can then be ruled out. These experiments will therefore provide important insights in any case.
Original publication:
N. Ahmadiniaz et al: Detection schemes for quantum vacuum diffraction and birefringence, Phys. Rev. D 108, 076005
DOI: 10.1103/PhysRevD.108.076005
https://journals.aps.org/prd/abstract/10.1103/PhysRevD.108.076005
Readers comments