Atomic Physics
The sun in the laboratory
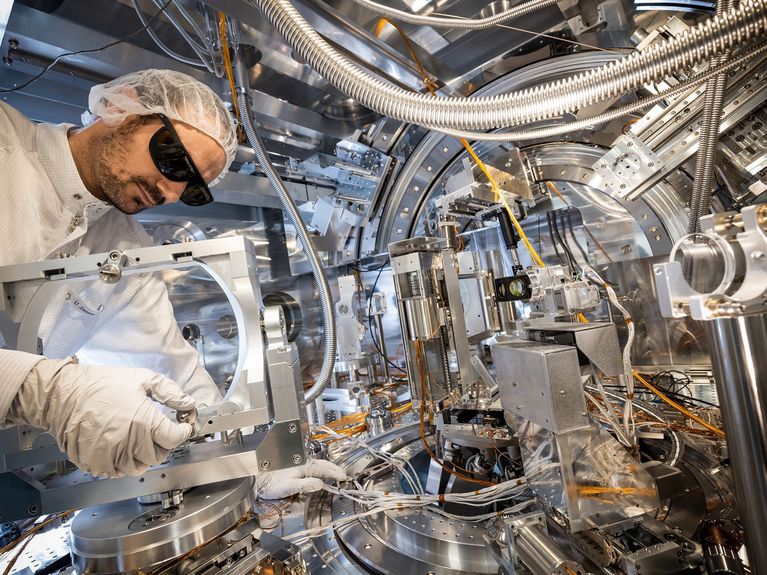
Laboratory at the Helmholtz International Beamline for Extreme Fields (HIBEF). Image: European XFEL/Jan Hosan
An international research team has succeeded in creating conditions in the laboratory as extreme as those that prevail inside stars. The experiments provide important insights for understanding suns and large planets.
In the lecture notes of the famous Fermi Lectures, given by the Italian nuclear physicist Enrico Fermi in the early 1950s, there is a diagram that is still known among physicists today. Handwritten and somewhat difficult to decipher, the note shows the states of matter in our universe as a function of temperature and pressure. From liquids and gases known to us, as we find them at low and higher energy densities on earth and which are seen universally rather the exception, to states that can be found in our sun, up to hot neutron gases and neutron stars.
The physicist Enrico Fermi sketched the states of matter of the universe in a simple graphic. The region of warm dense matter still puzzles us today. Graphic (edited): Facsimile reproduction of Enrico Fermi's notes for a lecture he gave in 1951-52. Source: Fermi, Enrico (1966): Notes on thermodynamics and statistics, p. 171.
At one point in the middle of Fermi's diagram, there is an area about which little is known. "This is the area we are interested in," says Dominik Kraus, Professor of Experimental Physics at the University of Rostock and Senior Scientist at the Helmholtz-Zentrum Dresden-Rossendorf (HZDR). "It encompasses so-called warm density matter, a very difficult area to penetrate in physical terms, both experimentally and theoretically, so far virtually terra incognita." At the high temperatures and pressures that prevail here in the region of warm dense matter a variety of elements are found in planets.
Dominik Kraus. Photo: private
Using very intense laser radiation, the research team to which Kraus belongs has succeeded in creating the extreme conditions of warm dense matter in the laboratory for trillionths of a second. Much like a hammer blow on an anvil generates enormous energy and high pressure to form wrought iron, the extremely focused, pulsed light from a laser fired at a small matter capsule creates the effect. The team was able to generate temperatures of millions of degrees Celsius and pressures of billions of atmospheres for fractions of a second and measure the effects on matter with X-rays.
"X-rays of unprecedented intensity, quality and brilliance on extremely short time scales open up the option of penetrating the high energy densities in matter under such extreme conditions in the first place," says Kraus. The examination capsules, in which substances such as beryllium are compressed by the laser light to 30 times their original density, are tiny, a mere two millimeters in diameter. "But inside them, we can precisely measure the basic parameters of the compressed matter, such as temperature and density," says Kraus.
Ronald Redmer. Photo: private
The analysis of the measurement data obtained under these conditions is correspondingly complex. "When you get into the realm of warm dense matter, you face the problem of modeling what happens to matter there, which becomes very challenging," explains Ronald Redmer, theoretical physicist and professor at the University of Rostock. "We're dealing with so-called many-particle problems and, given the extreme conditions, specific quantum effects that we can't describe by means of known approximation methods of quantum mechanics." However, advances in the field of computer technology with increasingly powerful processors and new computational methods, "machine learning" and artificial intelligence are opening up new possibilities.
The current results not only deepen our understanding of basic research, they also have implications for research into so-called inertial fusion, in which the fusion of, for example, heavy hydrogen is used to generate energy. Only last December, a proof-of-principle experiment at NIF successfully demonstrated for the first time that this principle, on which the hydrogen bomb is also based, can also be used for peaceful energy production, at least to some extent. The measurements of Dominik Kraus' team are also interesting for the field of astrophysics. "And this is where the Helmholtz International Beamline for Extreme Fields (HIBEF) at the European XFEL in Hamburg and Schenefeld comes into play," Kraus says. "The HIBEF is currently the only place in the world where both geophysics (low temperatures, high pressures) and plasma physics (high temperatures, low pressures) procedures can be performed in the same examination chamber." There, Kraus and Redmer's research team now plans to achieve conditions similar to those at NIF on a much smaller scale, which would allow for a much larger number of experiments than before. "We won't be able to achieve the very high energy densities there that we can at NIF," Kraus explains. "But we have the opportunity to reproduce states of matter like those inside smaller planets, states like those inside Earth or Uranus and Neptune, and maybe even like the physical fringes inside Jupiter or the sun."
Infographic: Laser- From cursiveness to innovation driver (DESY research magazine femto - Issue 01/23)
Many things still puzzle researchers. Why is the standard model of the sun not compatible with current measurements of the frequency distribution of the chemical elements? Why do we still not know exactly the melting curve of iron? Why do Uranus and Neptune, the twin ice planets of our solar system, behave so differently? How could the first stars have formed at all in the early days of the universe, when there were almost no heavy elements, which are essential in the early stages of any star formation? "With HIBEF and our research, we are bringing some of these fundamental questions into the laboratory, so to speak," says Dominik Kraus.
Publication: T. Döppner et al.: “Observing the onset of pressure-driven K-shell delocalization”. DOI: 10.1038/s41586-023-05996-8 https://www.nature.com/articles/s41586-023-05996-8
Readers comments