Water
The strangest liquid in the world
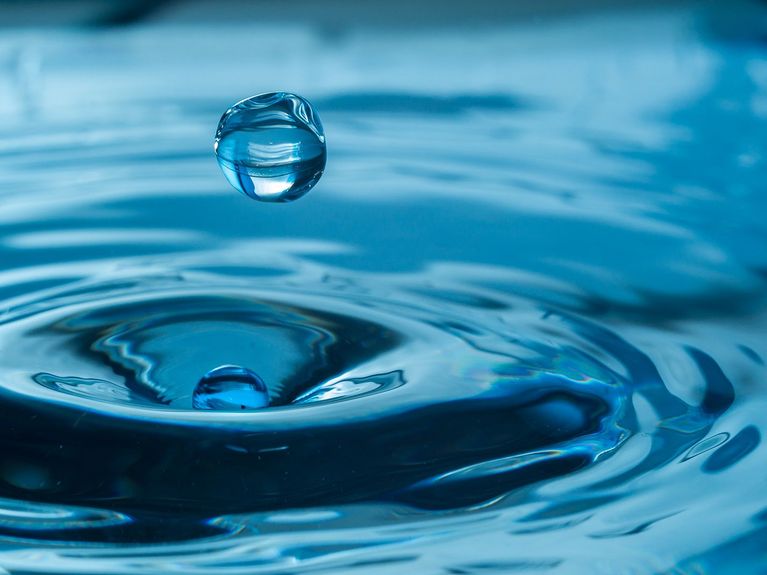
Picture: shutterstock / Peter Bocklandt
Water is commonplace. And yet many of its amazing properties, which are crucial for the emergence and maintenance of life, are still not properly understood. The interdisciplinary Centre for Molecular Water Science (CMWS) at DESY aims to change this.
Streams splash, waves roar, rain pelts, the bathroom tap drips. Water is a faithful companion; as a grandiose spectacle of nature, as the basis of all life, or even as a damp nuisance. In the eyes of science, however, H2O remains a mystery. Although it consists of only three atoms – two hydrogen, one oxygen – this simple constellation results in unusual properties; instead of whizzing around the room in gaseous form at room temperature like other comparable substances, water remains liquid in drinking cups and flower vases. Instead of becoming more viscous under high pressure, water becomes thin. Instead of sinking into the depths, icebergs float majestically across the polar seas. And without the water's own capillary forces, plants would not be able to supply themselves with nutrients.
Science now counts more than 50 of these water anomalies that are essential to our existence. "If water weren't so strange, we wouldn't exist," says Anders Nilsson from Stockholm University, one of the world's most renowned water researchers. Yet few of these anomalies are well understood and much basic research is still needed to unravel the properties and interactions of water molecules.
This research is to be bundled in a new, globally unique centre: Together with partners from all over Europe, DESY is planning to build the Centre for Molecular Water Science, or CMWS for short. It will shed light on the topic from a wide variety of disciplines: Physics, biophysics, medicine, climate research, astrochemistry, environmental technology.
Water is unusual
The anomalies are most noticeable when water is supercooled. Contrary to our everyday experience, H2O does not necessarily crystallize into ice at zero degrees Celsius. If it is highly pure, it can remain liquid even at sub-zero temperatures because there is a lack of condensation nuclei where the ice crystals form. The droplets of a cloud can therefore be as cold as minus 30 degrees Celsius.
Even in the laboratorycold water sometimes behaves strangely; unlike other substances, it can be compressed better the colder it is. Similarly with the heat capacity; the colder water is, the more heat it can absorb. Experts attribute these anomalies to the structure of the water molecule: Its "body" is formed by the oxygen atom, from which the two hydrogen atoms branch off as "little arms". The body tends to be negatively charged, whereas the arms are positively charged. This causes an attraction between the molecules; the negative body of one molecule is drawn to the positive arms of another and briefly forms a bond, the hydrogen bond.
Accordingly, water can be understood as a wafting network of molecules. These molecules constantly form spontaneous bonds with their neighbors, only to cut them off again immediately. Experts have long suspected that there are two different states of water depending on the number and orientation of the bridging bonds: A denser state (HDL = High-Density Liquid) and a less dense state (LDL = Low-Density Liquid). The density of HDL is 10 to 20 percent greater than that of LDL. So basically, water is made up of two different types. And then it would be the interplay of these two that is behind the anomalies.
A glass of water at room temperature is likely to consist mainly of the HDL phase, with tiny sprinkles of 20 to 30 molecules that temporarily combine to form the LDL phase. The problem is that the projections are so short-lived that new measuring techniques are needed to detect them. These techniques are to be developed at the CMWS to observe whether the mini-bubbles grow and become more stable at cooler temperatures, which would prove the HDL/LDL thesis correct.
Two counterparts called HDA (High-Density Amorphous Ice) and LDA (Low-Density Amorphous Ice) have already been discovered in certain glassy variants of water ice. In 2017, a team led by Anders Nilsson, DESY physicist Gerhard Grübel and Innsbruck ice researcher Thomas Lörting succeeded in a remarkable experiment: With the help of DESY's X-ray light source PETRA III, the working group was able to observe how HDA ice melted into HDL water at minus 150 degrees and rapid heating. For a tiny moment, this mutated into LDL water, only to solidify again into ice, this time in the LDA form.
For extremely low temperatures, the existence of both types of water thus seems to be confirmed. Now the final, conclusive proof is missing: The detection of HDL and LDL in liquid water.
Water in the X-ray laser
Some research teams follow the approach of adding salts to highly pure water in order to lower the freezing point in a controlled way without affecting the anomalous properties. Others rely on X-ray lasers such as the European XFEL in Hamburg. This generates ultra-short X-ray flashes that can analyze extremely rapid processes. In this way, water can be cooled very quickly and be observed to see exactly what is happening to it in the meantime.
One of the teams studying water using X-ray lasers is gathered around Gerhard Grübel, who is also one of the coordinators of the new Water Centre. In his laboratory stands the centerpiece of his experiment: A metal structure made of two intersecting vacuum tubes, reminiscent of a model of the space station. "A jet of the finest water droplets flies through one tube," Grübel explains. "Through the other one ultra-short X-ray flashes are shot through the droplets." The hope is that the droplets are so strongly supercooled that the coexistence of HDL and LDL will show up clearly when droplets and X-ray flashes meet in the intersection of the tubes. To do this, the droplets are specifically shot with the laser. "They then literally explode," says Grübel, "and we can observe this precisely with a high-speed camera." Although there are already many indications for the model with the two water phases, a direct verification is still missing. The European XFEL aims to provide it.
Water is life
H2O is of utmost importance for our existence; without water there is no life. Adults consist of about 50 to 65 percent water. Among other things, it determines the fluid balance in the body, dissolves solid foods such as sugar, salt and vitamins, and protects the body from overheating as a coolant. But it is also likely to play a central role in the body's cells. "However, we still know very little about what functions water fulfils there," says Henrike Müller-Werkmeister from the University of Potsdam. "We are only just beginning to get to grips with it."
Among other things, H2O seems to be important in regulating the pH value: Certain proteins pump protons (hydrogen nuclei) into or out of the cell. Short chains of water molecules are likely to act as regular climbing ropes in this process. Even when a protein molecule folds into its final shape after its formation, water mixes in vigorously, as experiments have shown.
Until now, however, it has hardly been possible to study such processes in detail. Protein crystallography, for example, in which strong X-ray light reveals the structure of biomolecules with atomic precision, works with deep-frozen crystallized samples because they can better withstand the intense radiation. But: "The dynamics of the water molecules in the proteins cannot be detected with this," Müller-Werkmeister explains. "The movement of the molecules is simply frozen."
A method developed mainly in Hamburg now provides a remedy: In serial femtosecond crystallography, the X-ray flashes no longer hit a frozen, large protein crystal, but a hair-thin beam of many small crystals. Because each of them is only hit once by the X-ray flash, cooling is unnecessary, meaning the experiments can run at room temperature, and the water network retains its realistic dynamics.
The new method has already given Müller-Werkmeister's team a surprise regarding the role of water when they used it to study an enzyme from a bacterium. A kind of molecular silent movie showed that the transmission of information between two subunits of the enzyme does not take place through a mechanical, quasi-gear-like action, as originally assumed, but via a "telephone line" consisting of four water molecules. "It is possibly a fundamental principle that such water networks regulate certain reactions of proteins," Müller-Werkmeister suspects. "We want to explore this in more detail in the future, especially within the framework of the new Water Centre at DESY."
Water in medicine
But there are other interactions between water and proteins that will be studied at the new Water Centre. Researchers at the Helmholtz Centre Dresden-Rossendorf (HZDR) want to find out how the water that coats the genetic material and other biomolecules directly influences their functions. They hope to discover new mechanisms that could be of use in medicine.
A research group led by Karim Fahmy, head of the Biophysics Department at the HZDR, is primarily investigating membrane proteins that are located on cell surfaces and transmit sensory perceptions and other signals, for example. When hormones or other active substances dock onto the cell receptors, this is communicated to the cell interior and triggers reactions. However, the water has a considerable influence on whether the docking maneuver works: "It is connected to the receptors via the hydrogen bonds, keeps them free to a certain extent, stabilizes the protein and helps the ligands, i.e., the binding substances, to position themselves," Fahmy explains. "On the other hand, the ligand has to bring a certain amount of energy to displace the water from the mooring site."
Karim Fahmy. Picture: HZDR
Knowing exactly how much energy is needed to do this and how the water influences it in detail would be of great advantage to the drug industry, for example, in order to develop new drugs, such as for the treatment of cancer. "One could prevent or promote the docking maneuvers to receptors in a more targeted way, depending on what helps a patient," says Fahmy.
These explosive functions of water were also decisive for the founding of a new research group in the Cluster of Excellence "Physics of Life" at TU Dresden, which is currently being set up by physical chemist Ellen Adams. Using a new technology at the HZDR, "terahertz spectroscopy", the researchers are investigating so-called intrinsically disordered proteins (IDPs) and their interaction with water. On time scales of a few picoseconds, which the new method makes possible, they observe how water and some other factors shape these dynamic proteins and thus determine their function. IDPs not only play a role in the processes of an intact body cell, but have also been shown to play a role in degenerative diseases such as Alzheimer's and ALS. This means that if we decipher the effects of water on IDPs, we may be able to specifically influence the structure of their products and thus prevent diseases.
Water in space
Other water experts are instead turning their gaze far away; into space. Space reveals an amazing chemical diversity. To date, telescopes have been able to identify more than 200 different types of molecules floating around, including complex compounds such as precursors of amino acids. But how did these form in interstellar space? In the almost pure vacuum between the stars, there are very few potential reaction partners wandering around, and they rarely meet. Moreover, it is bitterly cold, which makes chemical reactions more difficult.
The solution to the riddle probably has to do with water: "Today, we assume that a large part of the reactions there take place on the surfaces of ice particles," says DESY chemist Melanie Schnell. The idea is that over time the molecules encounter drifting ice grains and stick to them. There, stimulated by UV radiation, for example, they can react with other molecules that have also been captured. The resulting products can then evaporate again at some point.
Melanie Schnell. Picture: DESY
Some experts even speculate that some of the original building blocks of life have been created in this way. "With special experiments, for example, in a vacuum chamber in which we simulate the conditions of space, we are trying to find out how molecules form on such ice particles," reports Schnell, who is also the coordinator of the CMWS. Such experiments will be conducted at the new Water Centre. "They will possibly confirm our assumption that ice in space is a kind of interstellar catalyst."
Water to drink
Last but not least, the CMWS will also further develop technologies that meet our most basic need when it comes to water: To drink it. According to UNICEF, 2.2 billion people worldwide do not have regular access to clean water. That is why research is being done worldwide on technologies that could improve the situation. One source of hope is microporous polymer membranes. They can be used to remove even the most finely dispersed and dissolved pollutants from water. And they can desalinate seawater without having to heat it to 100 degrees.
Volkan Filiz's department at the Helmholtz Centre Hereon in Geesthacht is investigating such membranes. In principle, they function like a sieve and a magnet at the same time: "When we use them to filter polluted water, bacteria and viruses are held back due to their size while the water slips through," Filiz explains. "In addition, we can functionalize the membrane with quaternary ammonium compounds that bind pollutants such as heavy metals. Some heavy metals like arsenic and chromium are always negatively charged in water. That's why we make sure the membrane is positively charged and holds on to these pollutants through interactions." For many pollutants in water, the right materials and pore sizes are known to filter them out. Polymer membranes can also be used to effectively rid water of oil by using oil-repellent materials.
Membranes for the treatment of salt water into drinking water are not porous. They are as dense as cling film, but still contain nanometer-sized gaps through which the small water molecules fit, whereas salts do not. "However, you have to press the water through the membrane with a lot of pressure," admits Filz. Nevertheless, the energy input is lower than with conventional seawater desalination, for which the water is distilled with heat and the water vapor is collected. "Currently we are looking for the most energy-efficient combination of membrane and distillation processes." This so-called membrane distillation then works in principle like a Gore Tex jacket: It does not let water through, but the water vapor produced by heat does.
One of the main reasons why such membranes have not long since become established worldwide is their short shelf life. Wherever they are used as water filters, a biofilm forms over time that breaks them down. "Reducing this so-called fouling is one of our most important fields of research," says Filiz. The aim is to increase the service life of the membrane and thus improve its economic efficiency. Great hopes are pinned on polydopamine here. This is the natural adhesive with which mussels strongly adhere to rocks under water. Applied to a membrane, it has a hydrophilic effect; it interacts readily with water but repels foreign matter.
In order to develop optimal filters for a wide variety of purposes, researchers need to understand the interfacial effects between the polymers and the water in detail. This requires, not least, investigations at the atomic level, which the large-scale research facilities of the Helmholtz Association can provide. The CMWS Water Centre will bundle this research, attract water experts from all over the world and network them with each other. "Water is one of the key topics for the future," says Anders Nilsson. "The centre will enable us to decisively deepen our knowledge of it."
Readers comments