Nuclear Physics
Atomic nuclei set the pace
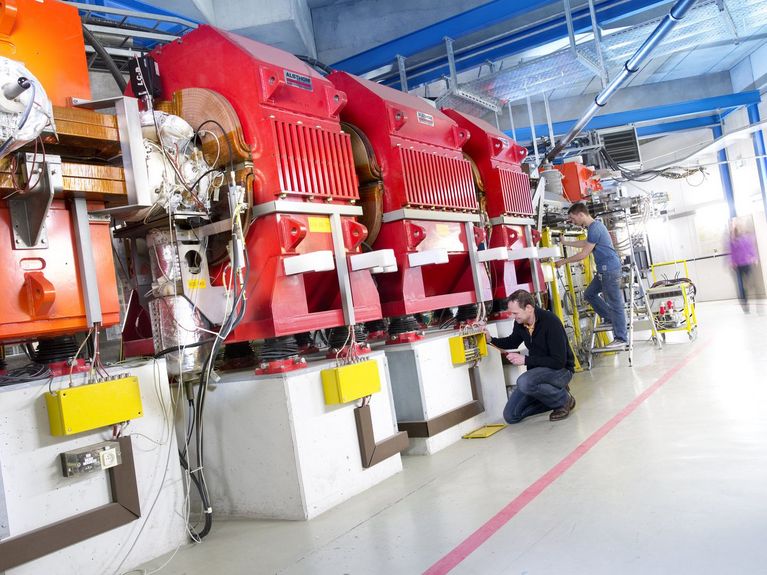
The investigations will take place at the GSI experimental storage ring ESR as part of HITHOR. Image: J. Hosan, HA Hessen Agentur
Ultra-precise nuclear clocks are the goal of Thomas Stöhlker’s research group. He has now received an Advanced Grant from the European Research Council for his new approach. The researchers want to use it to search for novel phenomena in particle physics.
They are set to revolutionize metrology: So-called nuclear clocks use atomic nuclei as timers, or more precisely, they use extremely stable electromagnetic oscillations required to excite an atomic nucleus to a higher level and thus complete a nuclear transition. It is hoped that this will enable even more precise time measurement compared to the atomic clocks in use today. Such precise clocks could be used to investigate whether the constants of nature are really as constant as thought. These and many other experiments require extreme precision, which is why the development of measurement technology is crucial for physical research.
However, it is by no means easy to build such a nuclear clock: Almost all nuclear transitions are at much too high energies and are therefore technically unsuitable for the construction of a clock. However, one isotope of the element thorium, thorium-229, has a transition that lies in the range of ultraviolet radiation and can be excited with the help of special lasers. This is why numerous research teams around the world are working on such a thorium nuclear clock
Thomas Stöhlker, Head of the Atomic, Quantum and Fundamental Physics research field at GSI/FAIR, Director of the Helmholtz Institute Jena and Professor at the Friedrich Schiller University Jena, has been awarded an ERC Advanced Grant by the European Research Council (ERC). Photo: HI Jena
Thomas Stöhlker and his working group now want to create a completely new approach to this as part of the HITHOR project (“Highly Ionized Trapped 229-Thorium: A New Paradigm Towards a Nuclear Clock”). He has now been awarded an ERC Advanced Grant from the European Research Council for this project. Stöhlker is Head of the Atomic, Quantum and Fundamental Physics Research Division at GSI/FAIR near Darmstadt, as well as Director of the Helmholtz Institute Jena and Professor at Friedrich Schiller University Jena. He says: “Our idea is to trap individual highly ionized thorium ions in an electromagnetic trap and work there with precision laser spectroscopy.” The trick is that the thorium ions should be extremely strongly ionized in these experiments, stronger than in any other such experiments.
“We have unique possibilities at GSI’s ESR ion storage ring, thanks to which we can remove almost all electrons from the thorium atoms,” says the physicist. To do this, the facility first accelerates uranium ions to more than 85 percent of the speed of light, which are partially converted to thorium-229 in an aluminum block during nuclear fragmentation – i.e., shattering due to the high energy – and are also stripped of most of their electrons. The atoms that have the desired number of only one or three electrons can then be selectively filtered out. These are then shot into the ESR at around 60 percent of the speed of light. There, the thorium ions can be slowed down and captured and stored in a trap in order to carry out precision laser spectroscopy.
“If such a thorium atomic nucleus is orbited by only one electron, we also speak of hydrogen-like thorium, because hydrogen, as the lightest element, naturally only has one electron,” says Stöhlker. However, thorium has 90 protons in its nucleus, so it lacks 89 electrons. This leads to an extremely strong electromagnetic interaction between the atomic nucleus and the electron. “Such unpaired electrons create a gigantic magnetic field at the nucleus, which broadens its energy levels,” explains Stöhlker. This is because this nuclear transition is normally extremely narrow. On the one hand, this is responsible for the high accuracy that is expected from nuclear clocks, but it also makes it extremely difficult to work with this transition.
Thanks to the strong ionization, the nuclear transition is widened by several orders of magnitude. “This has several pleasant effects,” explains Stöhlker. On the one hand, you don’t need an extremely narrow-band laser to be able to excite this nuclear transition with sufficient intensity. And on the other hand, you don't need as many thorium nuclei. “A common concept for a thorium nuclear clock is to fix the thorium atoms in solids, as otherwise there are too few atoms,” says the scientist. “But in our case, the transitions are much easier to excite, so we can work with just a few isolated atoms in an electromagnetic trap.”
This makes it possible to use the established methods of laser precision spectroscopy in ion traps and, among other things, to cool the thorium atoms down to extremely low temperatures using optical methods, lower temperatures than are possible in a solid state. “Such a nuclear clock would not only allow us to measure time with high precision, but also to search for new phenomena in nuclear and particle physics,” says Stöhlker. After all, it is still not understood why matter is predominant in our universe and not antimatter.
With the planned experiments, the team wants to shed new light on these questions. To this end, Stöhlker is planning several postdoctoral and doctoral positions, which are funded for five years as part of the project. “But we are also open to Bachelor's and Master’s students who want to take part,” concludes the researcher. In any case, the experiments promise a unique symbiosis of nuclear physics and laser spectroscopy and should open the door to a new era of metrology.
Readers comments